Top 8 Unexplained Phenomena In Physics That Leave Scientists Puzzled
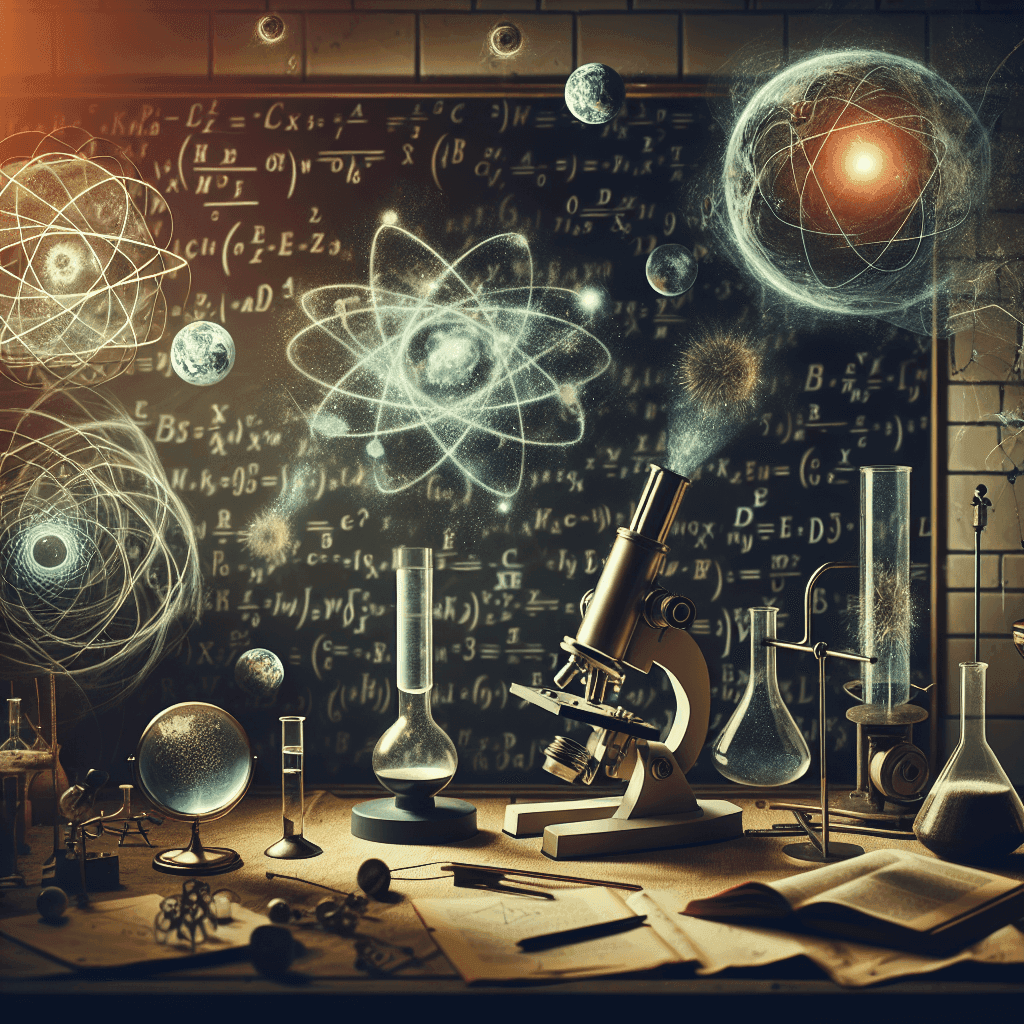
Throughout the history of physics, scientists have made remarkable discoveries that have expanded our understanding of the universe. However, there remain numerous phenomena that defy explanation, leaving researchers scratching their heads. These mysteries challenge the very foundations of our current knowledge and inspire ongoing inquiry and exploration. Here are the top eight unexplained phenomena in physics that continue to baffle scientists.
1. Dark Matter
Dark matter stands as one of the most enigmatic components of the universe, embodying a significant puzzle in the realm of modern physics. Despite constituting about 27% of the universe's total mass and energy, it remains invisible and undetectable through conventional means. Its existence is inferred primarily from gravitational effects on visible matter, radiation, and the large-scale structure of the cosmos. This mysterious substance defies direct observation, leading to a series of unanswered questions that challenge our understanding of fundamental physics.
The concept of dark matter emerged in the early 20th century when astronomers noticed discrepancies in the rotational speeds of galaxies. According to Newtonian physics and the visible mass of a galaxy, the outer stars should orbit more slowly than those closer to the center. However, observations revealed that stars at the edges of galaxies were moving at unexpectedly high speeds, suggesting there was more mass present than could be accounted for by visible matter alone. This discrepancy led to the hypothesis that an unseen form of matter, now termed dark matter, was exerting additional gravitational influence.
Despite extensive research, the nature of dark matter remains elusive. Various candidates have been proposed, ranging from weakly interacting massive particles (WIMPs) to axions and sterile neutrinos. Each of these hypotheses carries its own implications and challenges, and none have yet been confirmed through direct detection. Experiments designed to identify dark matter particles, such as those conducted in deep underground laboratories or through high-energy particle collisions, have yet to yield conclusive results. This ongoing search raises profound questions about the fundamental structure of matter and the forces that govern the universe.
Beyond dark matter, the universe is rife with other unexplained phenomena that baffle scientists. For instance, the accelerated expansion of the universe, attributed to dark energy, presents another layer of complexity. While dark matter seeks to explain the gravitational behavior of galaxies, dark energy accounts for the observed acceleration in cosmic expansion, making up about 68% of the universe. The interplay between these two dark components raises critical questions about the ultimate fate of the universe, the nature of gravity, and the potential for new physics beyond the standard model.
Another puzzling phenomenon is the behavior of black holes. These regions of spacetime, where gravity is so intense that nothing can escape, challenge our understanding of physics. The information paradox, which arises from the conflict between quantum mechanics and general relativity, remains unresolved. If information that falls into a black hole is lost forever, it contradicts the principle that information cannot be destroyed. This paradox highlights the limitations of our current theories and suggests that our understanding of fundamental physics is still incomplete.
Furthermore, the phenomenon of quantum entanglement poses intriguing questions about the nature of reality. In entangled systems, particles become linked such that the state of one instantly influences the state of another, regardless of the distance separating them. This "spooky action at a distance," as Einstein termed it, challenges classical intuitions about locality and causality, hinting at a deeper, underlying connection in the fabric of spacetime.
As scientists continue to grapple with these unexplained phenomena, the pursuit of knowledge remains a driving force in physics. Each discovery, whether confirming existing theories or challenging them, brings us closer to understanding the universe. Dark matter, with its profound implications for our comprehension of the cosmos, serves as a compelling reminder of the mysteries that still abound in the field of physics. The quest to unravel these mysteries not only expands our knowledge but also ignites curiosity about the fundamental laws that govern existence itself.
2. Dark Energy
Dark energy stands as one of the most perplexing and enigmatic concepts in modern physics, representing a significant portion of the universe's total energy density. Discovered in the late 1990s through observations of distant supernovae, dark energy is thought to be responsible for the accelerated expansion of the universe. Despite its prominence in cosmological models, the nature of dark energy remains shrouded in mystery, leaving scientists grappling with unanswered questions and unexplained phenomena.
The universe's expansion was initially expected to slow down due to gravitational attraction among galaxies. However, observations revealed that not only was the universe expanding, but the rate of this expansion was increasing. This unexpected acceleration led to the introduction of dark energy as a theoretical component, accounting for roughly 68% of the universe's energy content. Yet, what dark energy actually is continues to elude definitive explanation. Some theories propose that it could be a cosmological constant, a concept introduced by Albert Einstein, which suggests a uniform energy density filling space homogeneously. Others speculate it might be a dynamic field, known as quintessence, that evolves over time.
The challenge of understanding dark energy is compounded by the fact that it does not interact with matter in any conventional way. Unlike other forms of energy, such as electromagnetic or kinetic energy, dark energy does not emit light or radiation, making it invisible and detectable only through its gravitational effects on cosmic structures. This lack of direct evidence complicates efforts to study and understand its properties. Scientists are left to draw inferences from the large-scale structure of the universe, cosmic microwave background radiation, and the distribution of galaxies, but these methods yield only indirect insights.
Furthermore, dark energy poses philosophical and theoretical challenges. It raises questions about the fundamental nature of reality and the fabric of space-time. The concept stands in stark contrast to the established laws of physics, particularly in the realm of general relativity, where energy densities are expected to influence the curvature of space. The apparent disconnect between dark energy and established physical theories has led to a growing sense of unease among physicists, prompting some to explore radical new theories, including modifications to gravity itself.
In addition to dark energy, other unexplained phenomena in physics contribute to a growing sense of mystery in the field. For instance, dark matter, which constitutes about 27% of the universe, remains another elusive component. While dark energy is responsible for the expansion of the universe, dark matter is thought to provide the gravitational scaffolding that holds galaxies together. Despite extensive efforts, dark matter has yet to be directly detected, leading to a multitude of hypotheses ranging from weakly interacting massive particles (WIMPs) to more exotic candidates like axions.
Moreover, the phenomenon of cosmic inflation, which posits a rapid expansion of the universe in its earliest moments, adds another layer of complexity. The mechanisms behind inflation and how it relates to dark energy are still not fully understood, further complicating the narrative of cosmic evolution. Additionally, the fine-tuning problem raises questions about why the universe's expansion rate is so delicately balanced, suggesting that the conditions for life are incredibly specific and possibly improbable.
As scientists delve deeper into the cosmos, the interplay between dark energy, dark matter, and other unexplained phenomena continues to challenge our understanding of the universe. The quest to unravel these mysteries drives research in theoretical physics, cosmology, and astrophysics, pushing the boundaries of human knowledge. While dark energy remains a central enigma, the pursuit of answers may ultimately illuminate not just the cosmos, but also the fundamental principles that govern existence itself. In this ongoing journey, each discovery, no matter how small, brings us one step closer to understanding the profound mysteries that lie at the heart of the universe.
3. The Pioneer Anomaly
The Pioneer Anomaly presents a fascinating case of unexplained phenomena in physics that continues to intrigue scientists and enthusiasts alike. This anomaly refers to the unexpected deviation in the trajectories of the Pioneer 10 and Pioneer 11 spacecraft as they traveled through the outer regions of our solar system. Launched in the early 1970s, these spacecraft were designed for long-term missions to explore the outer planets and send valuable data back to Earth. However, as they ventured farther from the sun, both spacecraft exhibited an unanticipated acceleration toward the sun, a phenomenon that could not be readily explained by known gravitational forces or the influence of solar radiation.
Initially, scientists speculated that the anomaly could be attributed to various factors, including thermal radiation from the spacecraft itself or gravitational influences from unseen celestial bodies. The spacecraft generated heat from their onboard systems, and as this heat radiated away, it could have imparted a minute amount of thrust, potentially affecting their trajectories. However, after rigorous analyses and calculations, researchers concluded that these thermal effects alone could not account for the magnitude of the anomaly observed.
The Pioneer Anomaly raised fundamental questions about our understanding of gravity and the forces acting on spacecraft. It prompted physicists to explore alternative theories, including modifications to Newtonian gravity and the possibility of new physics beyond the established framework of general relativity. Some even speculated about the existence of undiscovered particles or forces that could be influencing the spacecraft's motion in ways not yet understood.
Despite extensive investigations, including a detailed study known as the “Pioneer Effect,” which involved analyzing the spacecraft's data over several years, a definitive explanation remained elusive. This uncertainty has led to an ongoing dialogue within the scientific community about the limits of our current theories and the potential for new discoveries that could arise from such anomalies. The Pioneer Anomaly serves as a reminder of the complexities of the universe and the challenges scientists face when confronted with data that does not conform to established theories.
In recent years, the anomaly has sparked renewed interest, as advancements in technology and data analysis techniques have opened new avenues for exploration. Some researchers have revisited the anomaly with fresh eyes, utilizing more sophisticated models and simulations to investigate the underlying physics. This renewed focus has not only deepened our understanding of the Pioneer missions but has also broadened the discussion to encompass a wider range of unexplained phenomena in physics.
The Pioneer Anomaly stands alongside other perplexing occurrences in physics, such as dark matter and dark energy, both of which challenge our comprehension of the cosmos. These phenomena highlight the limitations of our current models and underscore the necessity for continual inquiry and exploration. As scientists endeavor to understand the intricacies of the universe, the Pioneer Anomaly serves as a poignant reminder that there is still much to learn and discover.
In essence, the Pioneer Anomaly encapsulates the spirit of scientific inquiry, where the unexpected can lead to profound insights and potentially transformative breakthroughs. It invites both skepticism and curiosity, urging scientists to look beyond conventional wisdom and consider new possibilities. The quest to unravel the mystery of the Pioneer Anomaly and other unexplained phenomena in physics is not merely an academic pursuit; it is a journey that could reshape our understanding of the universe and our place within it. As research continues, the hope remains that the answers to such enigmas will one day illuminate the dark corners of our knowledge, revealing the underlying truths of the cosmos that have yet to be discovered.
4. Quantum Entanglement
Quantum entanglement stands as one of the most intriguing and perplexing phenomena in the realm of physics, captivating scientists and philosophers alike with its implications that challenge our conventional understanding of reality. This phenomenon occurs when pairs or groups of particles become interconnected in such a way that the state of one particle is directly related to the state of another, regardless of the distance separating them. If one particle is measured and its state determined, the other particle's state is instantaneously revealed, even if it is light-years away. This peculiar connection has led to a wealth of questions and discussions regarding the nature of information, causality, and the very fabric of spacetime.
The implications of quantum entanglement extend far beyond merely being a curious aspect of quantum mechanics; it raises fundamental questions about the nature of reality itself. Albert Einstein famously referred to entanglement as "spooky action at a distance," expressing his discomfort with the idea that information could travel faster than light. This skepticism underscores a broader philosophical debate that continues to resonate within the scientific community: Does entanglement suggest that our classical understanding of space and time is fundamentally flawed? Are there hidden variables that we have yet to discover, or does entanglement imply a more interconnected universe than previously thought?
As researchers probe deeper into the quantum realm, they encounter additional unexplained phenomena that further complicate their understanding. For instance, the concept of non-locality, which underpins entanglement, suggests that particles can influence one another instantaneously, defying traditional notions of locality where objects are only influenced by their immediate surroundings. This non-local behavior raises questions about the limits of causality and whether information can be transmitted beyond the constraints of space and time as we know them.
Moreover, experiments designed to test the predictions of quantum mechanics often yield results that challenge our intuitive understanding of reality. The famous Bell's theorem experiments have demonstrated that no local hidden variable theory can replicate the predictions of quantum mechanics, suggesting that entangled particles exhibit correlations that cannot be explained by any classical framework. This has led to a growing acceptance among physicists of the idea that our universe may be fundamentally non-local, a notion that continues to provoke debate and inquiry.
In the context of quantum technologies, entanglement has sparked advancements in quantum computing and quantum cryptography, harnessing the power of entangled states to create systems that outperform their classical counterparts. Yet, the operational principles of these technologies are rooted in a phenomenon that remains largely mysterious. While scientists have made significant strides in harnessing entangled states for practical applications, the underlying mechanisms and implications of entanglement continue to elude full comprehension.
In addition to its implications for technology, quantum entanglement also intersects with other unexplained phenomena in physics, such as dark matter and dark energy. Some researchers speculate that entanglement might play a role in these enigmatic components of the universe, potentially providing insights into their nature and how they interact with visible matter. The search for a unified theory that reconciles quantum mechanics with general relativity remains one of the greatest challenges in contemporary physics, and entanglement may hold clues that bridge these two fundamental pillars of our understanding.
As scientists delve deeper into the mysteries of quantum entanglement and its associated phenomena, they find themselves at the intersection of physics, philosophy, and even metaphysics. The questions raised by entanglement are not merely academic; they probe the very nature of reality, challenging our perceptions and prompting us to reconsider the principles that govern the universe. While quantum entanglement continues to baffle and inspire, it serves as a reminder of the vastness of our ignorance and the endless possibilities that lie within the fabric of reality, waiting to be unraveled. In this sense, entanglement is not just a phenomenon to be understood but a doorway to a deeper exploration of the universe’s most profound mysteries.
5. The Measurement Problem
The Measurement Problem stands as one of the most intriguing challenges within the realm of quantum mechanics, deeply intertwined with various unexplained phenomena that continue to baffle scientists. At its core, the Measurement Problem questions how and why quantum systems appear to exist in multiple states simultaneously, a concept encapsulated in the idea of superposition. When a measurement is made, these systems seem to "collapse" into one definitive state. This paradox raises profound questions about the nature of reality, observation, and the limits of human understanding.
For instance, consider the famous double-slit experiment, where particles such as electrons can display both wave-like and particle-like properties depending on whether they are observed. When unobserved, they exhibit interference patterns characteristic of waves, suggesting they traverse multiple paths simultaneously. However, the moment a measurement is taken to determine which path the particle takes, the interference pattern vanishes, and the particle behaves like a classical particle. This behavior challenges our intuitive understanding of reality, leaving scientists grappling with the implications of quantum mechanics on a macroscopic scale.
The Measurement Problem does not exist in isolation; it resonates with numerous unexplained phenomena across physics. One of the most prominent examples is the phenomenon of quantum entanglement, where particles become linked in such a way that the state of one particle instantly influences the state of another, regardless of the distance separating them. This "spooky action at a distance," as Einstein famously referred to it, defies classical notions of locality and causality. The implications of entanglement stretch beyond mere curiosity; they form the foundation of emerging technologies like quantum computing and quantum cryptography, yet they simultaneously deepen the mysteries surrounding the Measurement Problem.
Another perplexing area is the field of dark matter and dark energy, which together constitute about 95% of the universe's total mass-energy content. Despite their significant influence on cosmic structures and the expansion of the universe, dark matter and dark energy remain elusive, with scientists unable to directly observe or measure them. This lack of empirical evidence compounds the Measurement Problem, as it raises questions about how we define and measure the unseen components of our universe. If our current understanding of measurement is limited to observable phenomena, how do we account for the vast majority of the universe that defies direct observation?
The implications of the Measurement Problem also extend to the concept of reality itself. Some interpretations of quantum mechanics, such as the Copenhagen interpretation, suggest that reality is fundamentally probabilistic and dependent on observation, while other interpretations, like many-worlds theory, propose that all possible outcomes of a quantum event exist in a vast multiverse. These interpretations not only challenge our philosophical understanding of existence but also provoke debates about the nature of consciousness and its potential role in shaping reality.
The Measurement Problem and its associated unexplained phenomena highlight the limitations of our current scientific paradigms. As physicists strive to reconcile the strange behaviors of quantum systems with the classical world we experience, they are forced to confront questions that transcend empirical measurement. The ongoing search for a unified theory of quantum gravity, which seeks to integrate quantum mechanics with general relativity, may provide new insights into these perplexing issues, yet the road ahead remains fraught with challenges.
In conclusion, the Measurement Problem stands as a testament to the complexities and mysteries inherent in the fabric of our universe. It intertwines with various unexplained phenomena in physics, challenging our understanding of reality, observation, and the fundamental structure of existence. As scientists continue to explore these profound questions, they not only seek to unravel the enigmas of quantum mechanics but also to expand the boundaries of human knowledge in the face of the unknown. The journey promises to be as enlightening as it is perplexing, inviting future generations of physicists to ponder the very nature of reality itself.
6. The Nature of Time
The nature of time has long been a subject of fascination and perplexity within the realm of physics, particularly when explored in the context of unexplained phenomena that continue to baffle scientists. Time is often perceived as a linear progression, a continuous flow from the past through the present and into the future. However, various anomalies challenge this straightforward understanding, leading to profound questions about the very fabric of reality.
One of the most striking phenomena that raises questions about time is the concept of time dilation, a cornerstone of Einstein's theory of relativity. According to this theory, time does not pass at the same rate for everyone; it can stretch or contract depending on relative speeds and gravitational fields. This has been experimentally validated through observations of atomic clocks on fast-moving jets and satellites, which tick at different rates compared to those on Earth. While time dilation is well-documented, it leads to intriguing discussions about the subjectivity of time and how it might be experienced differently across various reference frames.
Another puzzling phenomenon is that of quantum entanglement, where particles become interconnected in such a way that the state of one instantly influences the state of another, regardless of the distance separating them. This instantaneous connection seems to defy the conventional understanding of time and space, suggesting that information can be transmitted faster than the speed of light, which contradicts the tenets of relativity. This raises deep questions about causality and the sequencing of events, prompting scientists to reconsider how time might operate at the quantum level.
Further complicating our understanding of time is the concept of time's arrow, which refers to the one-directional flow of time from past to future. This phenomenon is closely associated with the second law of thermodynamics, which states that entropy, or disorder, in a closed system tends to increase over time. While this provides a framework for understanding why time appears to move forward, it does not fully explain why we perceive time as flowing in one direction rather than another, leading to philosophical inquiries about the nature of reality itself.
Additionally, the role of black holes introduces further complexity into our understanding of time. The event horizon of a black hole presents a boundary beyond which nothing can escape, and time behaves oddly in this region. As one approaches a black hole, time appears to slow down dramatically relative to an outside observer. This raises questions about the ultimate fate of information and matter that crosses the event horizon, challenging our comprehension of time and causality in extreme gravitational contexts.
Moreover, theories such as loop quantum gravity and string theory propose that time might not even be a fundamental aspect of the universe but rather an emergent property arising from more basic components of reality. These theories suggest that at the most fundamental level, time could be woven into the very fabric of space itself, potentially existing in a state that is fundamentally different from our everyday experience.
As scientists endeavor to unravel these mysteries, the nature of time remains deeply intertwined with various unexplained phenomena in physics. Each breakthrough in understanding time not only sheds light on the universe's workings but also invites further questions about the nature of existence itself. The exploration of time, in all its complexity, continues to challenge our perceptions and assumptions, revealing a universe that is far more intricate and enigmatic than it appears at first glance. In a world where time seems both familiar and alien, the quest to understand it may ultimately lead to transformative insights about the cosmos and our place within it.
7. The Origin of Cosmic Rays
Cosmic rays are high-energy particles that travel through space and bombard the Earth’s atmosphere, originating from various sources in the universe. Their discovery dates back to the early 20th century, and since then, they have intrigued scientists due to their enigmatic nature and the unanswered questions surrounding their origins. Despite significant advancements in astrophysics, the precise mechanisms that produce cosmic rays remain a subject of ongoing research, leaving many puzzled.
One of the leading theories suggests that cosmic rays are generated by supernova explosions. When a massive star exhausts its nuclear fuel, it undergoes a catastrophic collapse, resulting in an explosion that expels material at incredible speeds. This violent process can accelerate particles to nearly the speed of light, creating the high-energy cosmic rays that reach Earth. However, while supernovae are a plausible source, they do not account for all observed cosmic rays, especially the highest energy ones.
Another potential source is active galactic nuclei (AGN), particularly those containing supermassive black holes. As matter spirals into these black holes, it heats up and emits intense radiation, potentially accelerating particles to high energies. Yet, the exact processes involved in this acceleration remain poorly understood, further complicating our understanding of cosmic rays. The presence of cosmic rays originating from AGN is suggested by their isotopic composition and energy distribution, but more evidence is needed to solidify this connection.
Pulsars, highly magnetized rotating neutron stars, are also considered as potential cosmic ray sources. They emit beams of electromagnetic radiation and can accelerate particles through their intense magnetic fields. The interplay of these magnetic fields and the pulsar's rotation could contribute to the acceleration of particles to relativistic speeds. However, the contribution of pulsars to the overall cosmic ray population is still debated among experts.
The search for the origins of cosmic rays is further complicated by the presence of ultra-high-energy cosmic rays (UHECRs), which possess energies exceeding those produced by human-made particle accelerators. These particles defy current understanding, as they appear to originate from astrophysical sources capable of producing such extreme energies. The mechanisms behind their acceleration, whether through shock waves in supernova remnants or interactions in other extreme environments, remain elusive.
Additionally, the detection of cosmic rays is hampered by their interactions with the Earth’s atmosphere. When cosmic rays collide with atmospheric particles, they create extensive air showers, making it challenging to trace their origins accurately. This complexity introduces a layer of uncertainty in determining the sources of cosmic rays, as the observed properties of the air showers can be influenced by various factors, including the energy of the incoming particles and the type of atmospheric interactions.
As scientists continue to investigate cosmic rays, they confront broader questions about the fundamental nature of the universe. The existence of cosmic rays hints at processes and phenomena that challenge our current understanding of physics. For instance, the acceleration mechanisms that propel these particles to such extraordinary speeds raise questions about the limits of particle physics and the conditions necessary for such events to occur.
The study of cosmic rays intersects with many unexplained phenomena in physics, including dark matter and high-energy astrophysical processes. Some researchers propose that cosmic rays might provide clues to the nature of dark matter, as their interactions could shed light on the elusive particles thought to make up a significant portion of the universe's mass. The quest to understand cosmic rays is thus intertwined with broader efforts to unravel the mysteries of the cosmos, further emphasizing the complex and interconnected nature of physical phenomena.
In summary, the origins of cosmic rays remain a captivating mystery that continues to challenge scientists. While various sources, including supernovae, active galactic nuclei, and pulsars, are proposed, the exact mechanisms of particle acceleration and the full range of cosmic ray origins are still not fully understood. As researchers delve deeper into these cosmic enigmas, they not only seek to uncover the secrets of cosmic rays but also to address fundamental questions about the universe itself, highlighting the profound connections between unexplained phenomena in physics and the quest for knowledge that drives scientific inquiry.
8. The Matter-Antimatter Asymmetry
The universe, as we know it, is predominantly composed of matter, yet theories in particle physics suggest that equal amounts of matter and antimatter should have been created during the Big Bang. This discrepancy, known as matter-antimatter asymmetry, presents one of the most perplexing puzzles in modern physics. While matter forms the stars, planets, and galaxies we observe, antimatter, which consists of particles that have the same mass as their matter counterparts but opposite charges, remains elusive. If equal amounts of both were produced, we would expect to observe significant amounts of annihilation events where matter and antimatter meet, resulting in high-energy gamma rays. However, the universe is largely devoid of observable antimatter, leading scientists to question the fundamental principles governing particle interactions.
Several theories have been proposed to address this asymmetry, with one of the most prominent being the concept of baryogenesis. This theory suggests that certain conditions in the early universe allowed for a slight excess of baryons (particles like protons and neutrons) over antibaryons. These conditions might have been influenced by processes that violate the symmetries of particle interactions, known as CP violation. While CP violation has been observed in certain particle decays, the extent of the violation is insufficient to account for the significant imbalance between matter and antimatter in the universe. This leaves scientists seeking alternative explanations or new physics that could provide insights into the origins of this asymmetry.
Another avenue of exploration involves the potential existence of new particles or forces that could alter our understanding of matter and antimatter interactions. Theories such as supersymmetry or extra dimensions propose the existence of particles beyond the Standard Model of particle physics, which could interact differently with matter and antimatter. These hypothetical particles might provide mechanisms for generating the observed asymmetry, but so far, experiments at particle accelerators like the Large Hadron Collider have not confirmed their existence.
Additionally, the role of neutrinos, the elusive particles that interact only via the weak nuclear force, has garnered attention in the search for answers. Neutrinos are known to oscillate between different types, which hints at a complex and poorly understood underlying physics. Some models suggest that the properties of neutrinos could be tied to the matter-antimatter imbalance, particularly in scenarios where lepton number violation occurs. This could lead to processes that preferentially produce matter over antimatter, but as with other theories, empirical evidence remains limited.
Cosmological observations also provide clues into the matter-antimatter enigma. The cosmic microwave background radiation, a relic from the early universe, shows a uniformity that could suggest a process that favored matter over antimatter. Furthermore, the distribution of galaxies and the large-scale structure of the universe might reflect the influence of this asymmetry, yet the exact mechanisms remain speculative.
As scientists delve deeper into the mysteries surrounding matter-antimatter asymmetry, they are continuously confronted with questions that challenge the very foundations of physics. The search for a definitive explanation not only aims to illuminate the origins of our universe but also to understand the fundamental laws that govern it. As technology advances and experimental techniques become more refined, the hope remains that these unexplained phenomena will eventually yield to human inquiry, leading to breakthroughs that could reshape our understanding of reality. The quest to unravel the matter-antimatter imbalance is a testament to the enduring spirit of scientific exploration, one that seeks to illuminate the dark corners of our universe and the fundamental forces that shape it.