Top 8 Amazing Facts About Quantum Mechanics
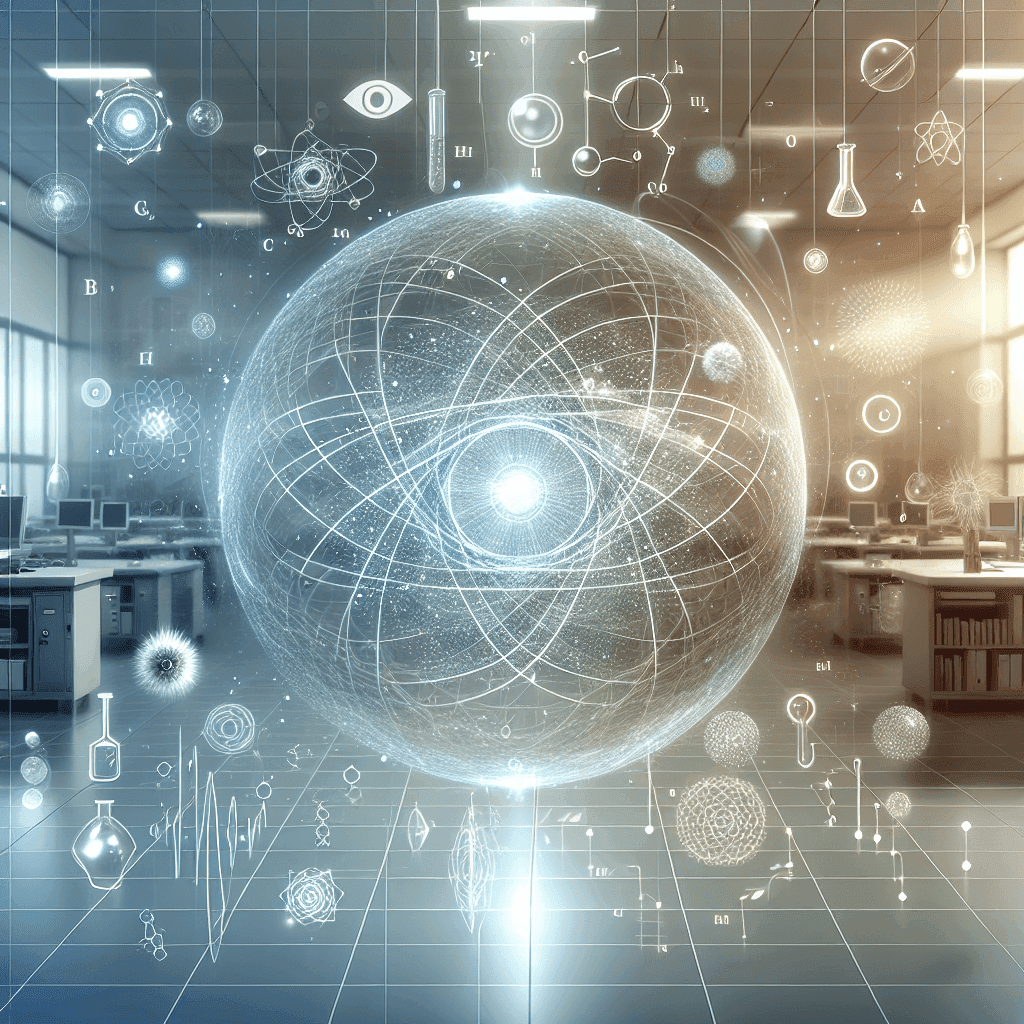
Quantum mechanics is a fascinating and complex branch of physics that delves into the behavior of matter and energy at the smallest scales. It challenges our classical intuitions and introduces concepts that can seem mind-boggling. Here are eight amazing facts that highlight the intriguing nature of quantum mechanics.
1. Wave-Particle Duality
Wave-particle duality is one of the most fascinating and perplexing concepts in quantum mechanics, capturing the imagination of scientists and laypeople alike. At its core, wave-particle duality suggests that particles, such as electrons and photons, exhibit both wave-like and particle-like properties, depending on the experimental conditions. This dual nature challenges our classical intuitions about the behavior of matter and energy, leading to remarkable implications for our understanding of the universe.
The concept emerged in the early 20th century, primarily through the work of physicists like Albert Einstein and Louis de Broglie. Einstein’s explanation of the photoelectric effect provided crucial evidence for the particle aspect of light, demonstrating that photons can be considered discrete packets of energy. Meanwhile, de Broglie proposed that all matter has wave-like characteristics, suggesting that electrons can be described as waves. This revolutionary idea opened the door to the development of quantum mechanics, fundamentally altering our perception of reality.
Experiments such as the double-slit experiment vividly illustrate wave-particle duality. When light or electrons are fired at a barrier with two slits, they create an interference pattern on a screen behind the barrier, characteristic of waves. However, if one attempts to measure which slit a particle goes through, the interference pattern disappears, and particles behave like classical objects, producing two distinct bands on the screen. This paradox raises profound questions about observation and reality, suggesting that the act of measurement fundamentally alters the system being observed.
Another astonishing aspect of wave-particle duality is its implications for entanglement and non-locality. When particles become entangled, the state of one instantly influences the state of another, regardless of the distance separating them. This phenomenon has been described as “spooky action at a distance” by Einstein himself, who was skeptical of the implications of quantum mechanics. The entangled particles exhibit a wave-like correlation, reinforcing the idea that their behavior transcends classical boundaries, further intertwining the wave and particle nature of reality.
In addition to its philosophical implications, wave-particle duality has practical applications in technology. Quantum mechanics, with its principles rooted in wave-particle behavior, has paved the way for innovations such as semiconductors, lasers, and quantum computing. These advancements rely on the fundamental understanding that particles can exist in superpositions of states, leading to unprecedented computational power and efficiency.
Moreover, the concept of wave-particle duality has inspired a wealth of research and exploration into the foundations of quantum mechanics. Scientists continue to investigate the implications of this duality, exploring areas such as quantum field theory and quantum gravity. As our understanding deepens, we are challenged to rethink the very nature of reality, pushing the boundaries of knowledge and imagination.
Wave-particle duality is a cornerstone of quantum mechanics, revealing a world that defies conventional logic and intuition. It embodies the strange yet beautiful nature of the quantum realm, where particles can behave like waves and vice versa, depending on how we choose to observe them. This duality not only revolutionizes our understanding of the universe but also invites us to embrace the mysteries of existence, encouraging a sense of wonder in the face of the unknown. The exploration of wave-particle duality, and its implications, continues to inspire scientists and thinkers, reminding us that the universe is far more intricate and interconnected than it appears, and that the quest for knowledge is a journey that transcends the boundaries of time and space.
2. Quantum Superposition
Quantum mechanics, the branch of physics that deals with the behavior of matter and energy at the smallest scales, is filled with concepts that challenge our classical intuitions. One of the most astonishing phenomena in this realm is quantum superposition, a principle that defies our everyday understanding of reality. To grasp the implications of superposition, one must first appreciate the foundational ideas of quantum mechanics, where particles like electrons and photons exist not as singular, well-defined entities but as probabilistic wave functions.
At the heart of quantum superposition is the idea that a quantum system can exist in multiple states simultaneously until it is measured. This means that a particle can be in various positions, energies, or even spin states all at once. A classic illustration of this concept is the thought experiment known as Schrödinger's cat. In this scenario, a cat is placed in a sealed box along with a radioactive atom, a Geiger counter, and a vial of poison. If the atom decays, the Geiger counter triggers the release of the poison, resulting in the cat's demise. Until someone opens the box to observe the cat, it is said to be in a superposition of being both alive and dead. This paradox underscores the strange reality of quantum mechanics, where observation plays a crucial role in determining the state of a system.
Superposition is not merely a theoretical curiosity; it has practical applications that are revolutionizing technology. Quantum computing, for instance, harnesses the power of superposition to process information in ways that classical computers cannot. While a classical bit can represent a 0 or a 1, a quantum bit, or qubit, can represent both 0 and 1 simultaneously. This capability enables quantum computers to perform complex calculations at speeds unattainable by their classical counterparts, offering the potential to solve problems in cryptography, optimization, and drug discovery that would take conventional computers millennia to tackle.
Moreover, superposition has implications beyond computing. In quantum cryptography, the principle is used to create secure communication channels. Because the act of measuring a quantum state can change it, any attempt to eavesdrop on a quantum communication line will inevitably disturb the information being transmitted. This ensures that the parties involved can detect any unauthorized access, providing a level of security that classical methods cannot match.
The phenomenon of superposition also plays a role in the field of quantum teleportation, a process by which the state of a quantum system can be transmitted from one location to another without moving the physical particle itself. This process relies on entanglement, another fascinating aspect of quantum mechanics, where particles become interconnected in such a way that the state of one particle instantaneously influences the state of another, regardless of the distance separating them. Through the combination of entanglement and superposition, quantum teleportation exemplifies the interconnectedness of quantum systems and challenges our comprehension of space and time.
As researchers delve deeper into the mysteries of quantum mechanics, the implications of superposition continue to inspire both wonder and skepticism. It forces us to reconsider our understanding of reality, suggesting that the universe at its most fundamental level is far more complex and interconnected than we can perceive. While quantum mechanics may seem esoteric and abstract, its principles are increasingly shaping the future of technology and our understanding of the universe. The captivating nature of quantum superposition serves as a reminder that the building blocks of reality are not as fixed as they seem, opening the door to possibilities that stretch the limits of human imagination.
Read More: Top 8 Most Influential People of All Time
3. Quantum Entanglement
Quantum mechanics, the branch of physics that deals with the behavior of matter and energy at the smallest scales, often challenges our intuitive understanding of the universe. Among its most astonishing phenomena is quantum entanglement, a concept that not only captivates scientists but also intrigues philosophers and enthusiasts alike. This peculiar phenomenon occurs when particles become interconnected in such a way that the state of one particle is directly related to the state of another, no matter how far apart they may be. This baffling relationship raises profound questions about the nature of reality and the limits of information transfer.
To grasp the essence of quantum entanglement, one must first understand the principle of superposition. In the quantum realm, particles can exist in multiple states simultaneously until they are measured. This is exemplified by Schrödinger's famous thought experiment involving a cat that is both alive and dead until observed. When two particles become entangled, their states are intertwined in such a manner that measuring one particle instantly determines the state of the other, regardless of the distance separating them. This phenomenon was famously dubbed "spooky action at a distance" by Albert Einstein, who was skeptical of the implications it held for the nature of reality.
One of the most remarkable aspects of entanglement is its role in quantum teleportation, a process that allows the transfer of quantum information from one particle to another without physically moving the particle itself. This is achieved by entangling a pair of particles, then measuring one of them, which instantaneously affects the state of the other. Although this does not involve the transportation of matter as depicted in science fiction, it demonstrates the potential for instantaneous communication of information across vast distances, challenging our classical notions of locality and causality.
Entanglement has practical applications that extend beyond theoretical physics. It is a cornerstone of quantum computing, where entangled qubits can perform calculations at unprecedented speeds compared to classical bits. This ability to process vast amounts of information simultaneously makes quantum computers poised to revolutionize fields such as cryptography, materials science, and complex system modeling. Furthermore, quantum entanglement is essential for the development of quantum networks, which aim to create ultra-secure communication channels by leveraging the principles of quantum mechanics.
Moreover, experiments have consistently confirmed the predictions of quantum mechanics regarding entanglement. Notable tests, such as those inspired by Bell's theorem, have shown that entangled particles violate classical notions of separability and local realism, indicating that the universe at its core is interconnected in ways that defy our everyday experiences. These experiments have not only validated the existence of entanglement but have also sparked philosophical debates about determinism, free will, and the very fabric of reality.
The implications of quantum entanglement extend into the realms of philosophy and metaphysics, prompting discussions about the nature of consciousness and the interconnectedness of all things. Some interpretations of quantum mechanics suggest that entanglement could play a role in understanding phenomena such as consciousness and awareness, leading to intriguing hypotheses about the relationship between the observer and the observed.
As research in quantum mechanics continues to evolve, the enigma of entanglement remains at the forefront of scientific inquiry. It challenges our traditional concepts of space, time, and information, inviting us to reconsider what we know about the universe. Whether through its potential applications in technology or its philosophical implications, quantum entanglement stands as a testament to the extraordinary and often counterintuitive nature of the quantum world, reminding us that reality is far more complex and interconnected than it appears at first glance.
4. The Observer Effect
The Observer Effect is one of the most fascinating and perplexing concepts in quantum mechanics, intertwining the realms of physics and philosophy in a way that challenges our understanding of reality. At its core, the Observer Effect suggests that the act of observation can influence the behavior of quantum systems. This phenomenon emerges from the peculiar nature of particles at the quantum level, where they exist in a state of superposition, embodying multiple possibilities simultaneously until they are measured or observed.
To grasp the implications of the Observer Effect, one must delve into the famous double-slit experiment, which illustrates the strange behavior of particles such as electrons and photons. When particles are fired at a barrier with two slits, and no measurement is made, they create an interference pattern on a screen behind the barrier, indicative of wave-like behavior. However, when an observer attempts to measure which slit the particles pass through, the interference pattern collapses, and the particles behave as if they are solid objects, producing two distinct bands. This striking shift underscores how the act of measurement alters the outcome, suggesting that consciousness or the act of observation plays a crucial role in determining the state of reality.
The implication of the Observer Effect extends beyond mere observation; it raises profound questions about the nature of existence itself. If the act of observing can change the state of a quantum system, what does that mean for the reality we perceive? Are we, as observers, co-creators of the universe, shaping the fabric of reality with our consciousness? This notion has led to various interpretations of quantum mechanics, including the Copenhagen interpretation, which posits that quantum particles do not have definite properties until they are measured. In contrast, other interpretations, such as the Many-Worlds interpretation, suggest that all possible outcomes occur, branching out into parallel realities whenever an observation is made.
Moreover, the Observer Effect has significant implications for technology and our understanding of the universe. Quantum computing, for instance, relies on the principles of superposition and entanglement, where quantum bits can exist in multiple states simultaneously. However, the Observer Effect poses challenges in maintaining coherence in these systems, as any attempt to measure the qubits can disrupt their delicate states. This interplay between observation and quantum states is a critical area of research, as scientists strive to harness the power of quantum mechanics for advanced computing and secure communication.
In a broader sense, the Observer Effect invites us to reconsider our relationship with the universe. While classical physics presents a deterministic view of reality, quantum mechanics introduces an element of unpredictability and interconnectedness. The idea that our observations can influence the behavior of particles suggests a deeper unity between the observer and the observed, echoing philosophical inquiries that have persisted throughout human history. The implications of the Observer Effect extend beyond the confines of laboratories and theoretical physics, prompting us to explore the nature of consciousness, reality, and our place within the cosmos.
In conclusion, the Observer Effect serves as a gateway to understanding the intricate and often bewildering world of quantum mechanics. It challenges our perception of reality, blurring the lines between observer and observed, and invites us to ponder the fundamental nature of existence. As researchers continue to explore the depths of quantum phenomena, the Observer Effect remains a captivating reminder of the mysteries that lie at the heart of the universe, urging us to embrace the enigma of our reality with curiosity and wonder.
5. Heisenberg's Uncertainty Principle
In the realm of quantum mechanics, few concepts are as intriguing and mind-bending as Heisenberg's Uncertainty Principle. Formulated by the German physicist Werner Heisenberg in 1927, this principle fundamentally alters our understanding of the behavior of particles at the quantum level. It posits that certain pairs of physical properties, known as complementary variables or conjugate variables, cannot both be precisely measured at the same time. The most famous example is the relationship between a particle's position and its momentum. The more accurately we know a particle's position, the less accurately we can know its momentum, and vice versa. This intrinsic limitation is not merely due to experimental imperfections but is a fundamental characteristic of nature itself, illustrating the bizarre reality of the quantum world.
The implications of the Uncertainty Principle extend far beyond mere measurement limitations. They challenge our classical intuitions about reality. In the classical world, we can measure position and momentum simultaneously with arbitrary precision, but in the quantum realm, this certainty evaporates. This principle implies that particles do not have definite positions or momenta until they are measured. Instead, they exist in a state of probability, described by a wave function that encodes all possible outcomes. The act of measurement collapses this wave function into a specific state, forcing the particle to "choose" its position or momentum, but not both. This introduces a fundamental randomness into the behavior of particles, making quantum mechanics inherently probabilistic rather than deterministic.
Heisenberg’s Uncertainty Principle has profound philosophical implications as well. It invites us to reconsider the nature of reality itself. If we cannot know both position and momentum with certainty, what does that say about our understanding of the universe? It raises questions about the very essence of existence and the limits of human knowledge. Some interpretations of quantum mechanics, such as the Copenhagen interpretation, suggest that particles do not have definite properties until they are observed, while others propose that the universe exists in a state of superposition, where multiple outcomes can occur simultaneously. This challenges our classical notions of causality and determinism, suggesting instead that the universe is a complex tapestry of probabilities.
In addition to its philosophical ramifications, the Uncertainty Principle has practical applications in modern technology. Quantum mechanics, and by extension the Uncertainty Principle, underpins many technologies that are integral to our daily lives, such as semiconductors and lasers. The development of quantum computing, a field that harnesses the principles of quantum mechanics, is another exciting frontier. Quantum computers leverage the superposition and entanglement of quantum states to perform calculations at speeds unimaginable with classical computers. The uncertainty inherent in quantum states can be seen as a source of computational power that could revolutionize fields ranging from cryptography to complex system simulations.
Moreover, the Uncertainty Principle has been experimentally confirmed in numerous ways, including through the behavior of electrons in atoms and the properties of photons. The phenomenon of quantum tunneling, where particles pass through potential barriers that they classically shouldn't be able to surmount, is another manifestation of the principles outlined by Heisenberg. This has real-world implications, such as in nuclear fusion, where particles must tunnel through energy barriers to fuse together, a process that powers the sun and other stars.
The amazing facts about quantum mechanics, highlighted by Heisenberg's Uncertainty Principle, continually astonish and inspire scientists and philosophers alike. As we delve deeper into the quantum realm, we uncover a world that is both beautiful and perplexing, filled with mysteries that challenge our deepest assumptions about reality. The Uncertainty Principle serves as a gateway into this extraordinary domain, reminding us that at the heart of the universe lies an intricate dance of probabilities that defies our classical understanding. In embracing the uncertainty, we not only expand our knowledge of the physical world but also enrich our appreciation for the complexities of existence itself.
6. Quantum Tunneling
Quantum mechanics, the branch of physics that delves into the behavior of matter and energy at the smallest scales, is replete with astonishing phenomena that challenge our classical intuitions. One of the most intriguing aspects of quantum mechanics is quantum tunneling, a process that not only highlights the peculiar nature of the quantum world but also has profound implications for various scientific fields, including chemistry, physics, and even technology.
At its core, quantum tunneling refers to the ability of a particle to pass through a potential energy barrier, even when it seemingly lacks the energy to do so according to classical physics. Imagine rolling a ball up a hill: if the ball doesn't have enough energy to reach the top, it simply rolls back down. However, in the quantum realm, particles such as electrons behave like waves and are described by probability functions. This means that there is a non-zero probability of the electron being found on the other side of the barrier, even if it doesn't have the energy to overcome it in the traditional sense. The wave-like nature of particles allows them to "tunnel" through barriers, emerging on the other side as if they had never encountered an obstacle.
This phenomenon might seem purely theoretical, yet it has been observed and measured in numerous experiments. One of the most famous examples of quantum tunneling is in nuclear fusion, the process that powers the sun. In the sun's core, hydrogen nuclei must overcome a significant energy barrier to collide and fuse into helium. Classical physics would suggest that the temperatures and pressures in the sun are insufficient for this to occur. However, due to quantum tunneling, hydrogen nuclei can occasionally tunnel through the barrier, allowing fusion to take place and releasing the energy that fuels our solar system.
Moreover, quantum tunneling plays a crucial role in many chemical reactions. Enzymes, the biological catalysts that facilitate biochemical reactions, often rely on tunneling to speed up processes that would otherwise take an impractically long time. For instance, in some cases, protons can tunnel through energy barriers in enzymatic reactions, allowing for rapid transformations that are essential for life. This has led scientists to reconsider the mechanisms of enzymatic action and the fundamental principles governing chemical reactions.
The implications of quantum tunneling extend beyond natural processes; they have also inspired technological advancements. Quantum tunneling is a fundamental principle behind devices such as tunnel diodes and scanning tunneling microscopes. Tunnel diodes exploit the tunneling effect to achieve rapid switching speeds, making them invaluable in high-frequency applications. Scanning tunneling microscopes, on the other hand, utilize the tunneling of electrons between a sharp tip and a conductive surface to create atomic-scale images, revolutionizing our understanding of materials at the nanoscale.
In addition to its applications, quantum tunneling challenges our philosophical understanding of reality. It raises questions about determinism and the nature of existence itself. In classical physics, particles have well-defined positions and velocities; in quantum mechanics, however, particles exist in a superposition of states until observed. This means that the act of observation itself influences the outcome, leading to the perplexing realization that reality at the quantum level is fundamentally probabilistic rather than deterministic.
As we continue to explore the mysteries of quantum mechanics, quantum tunneling stands out as a testament to the strange and counterintuitive nature of the universe. It reveals that the boundaries of possibility are not as rigid as they seem and that the microcosm of particles behaves in ways that can defy our most basic expectations. This phenomenon not only enriches our understanding of the physical world but also paves the way for future innovations, inviting us to rethink what is possible in both science and technology. The marvels of quantum mechanics, exemplified by quantum tunneling, remind us that the universe is far more intricate and fascinating than we could ever have imagined.
7. The Quantum Zeno Effect
Quantum mechanics, the fundamental theory that describes the physical properties of nature at the scale of atoms and subatomic particles, is replete with astonishing phenomena that challenge our classical intuitions. One of the most intriguing of these phenomena is the Quantum Zeno Effect, a concept that exemplifies the strange and counterintuitive nature of quantum behavior. At its core, the Quantum Zeno Effect illustrates how the act of observation can influence the state of a quantum system, leading to outcomes that seem paradoxical from a classical perspective.
In essence, the Quantum Zeno Effect posits that a quantum system can be prevented from evolving into a different state if it is measured frequently enough. This effect derives its name from Zeno of Elea, a philosopher who proposed paradoxes that questioned the nature of motion and change. In the quantum realm, the effect manifests in a way that suggests continuous observation can "freeze" a quantum system in its current state. For example, consider a particle that has a probability of decaying over time. If we observe this particle at very short intervals, the decay process can be inhibited, essentially keeping the particle in a "non-decayed" state longer than it would have remained otherwise.
This phenomenon raises profound questions about the nature of reality and the role of the observer in quantum mechanics. In classical physics, the act of measurement is often seen as a passive process that reveals pre-existing properties of a system. However, in the quantum world, measurement plays an active role, influencing the state of the system itself. This interplay between observation and reality is a cornerstone of quantum mechanics, leading to a deeper understanding of the fabric of the universe.
The Quantum Zeno Effect has been experimentally demonstrated in various settings, where researchers have observed particles being effectively "frozen" in their states through rapid measurement. For instance, experiments with trapped ions and photons have shown that frequent measurements can significantly alter the behavior of these quantum entities, providing a tangible manifestation of this otherwise abstract concept. Such findings not only underscore the bizarre nature of quantum mechanics but also open up potential applications in quantum computing and information technology, where controlling quantum states is crucial for the development of robust quantum systems.
Moreover, the Quantum Zeno Effect invites us to reconsider our philosophical interpretations of quantum mechanics. It suggests a reality that is dynamic and interconnected, where the observer cannot be divorced from the observed. This interplay raises questions about determinism and free will, as the act of observation itself can dictate the evolution of a quantum system. In this light, the Quantum Zeno Effect serves as a reminder that our understanding of the universe is still evolving, and that the fundamental nature of reality may be far more intricate than we can currently comprehend.
As we delve deeper into the implications of the Quantum Zeno Effect, we find it nestled among other remarkable aspects of quantum mechanics, such as superposition, entanglement, and wave-particle duality. Each of these phenomena challenges our conventional understanding and invites us to explore the universe through a lens that embraces uncertainty and complexity. The Quantum Zeno Effect, with its paradoxical implications, is a testament to the strange yet beautiful world of quantum mechanics, where the act of observation can transcend mere measurement and shape the very fabric of reality itself. In a universe governed by quantum laws, the boundaries of what we perceive as possible are continually redrawn, leading us to a deeper appreciation of the mysteries that lie at the heart of existence.
8. Quantum Computing Potential
Quantum computing is often heralded as the next frontier in technology, promising to revolutionize fields ranging from cryptography to drug discovery. At the heart of this potential lies the fascinating world of quantum mechanics, a realm where the rules of classical physics break down and the bizarre becomes the norm. Understanding the interplay between quantum mechanics and computing unveils a treasure trove of amazing facts that underscore the transformative power of quantum technologies.
One of the most astonishing aspects of quantum mechanics is the phenomenon of superposition. In classical computing, a bit is either a 0 or a 1, serving as the basic unit of information. In contrast, a quantum bit, or qubit, can exist simultaneously in a state of 0, 1, or any quantum superposition of these states. This ability allows quantum computers to process vast amounts of information at once, exponentially increasing their computational power. For instance, while a classical computer would need to perform calculations sequentially, a quantum computer can tackle multiple possibilities in parallel, making it particularly suited for complex problem-solving tasks.
Entanglement, another cornerstone of quantum mechanics, offers a glimpse into the potential of quantum computing. When qubits become entangled, the state of one qubit is intrinsically linked to the state of another, regardless of the distance separating them. This interconnectedness allows quantum computers to perform operations that would be impossible for classical systems. The implications for communication and data security are profound; entangled qubits could lead to unbreakable encryption methods, ensuring the integrity of information in an increasingly digital world.
The concept of quantum tunneling adds yet another layer of intrigue to the potential of quantum computing. In classical physics, an object must have enough energy to overcome barriers in its path. However, in the quantum realm, particles can tunnel through barriers, appearing on the other side without having to surmount the obstacle in a traditional sense. This principle could enable quantum computers to explore solution spaces far more efficiently than their classical counterparts, particularly in optimization problems where the number of potential configurations can be astronomical.
As researchers delve deeper into the capabilities of quantum computing, they are also uncovering its potential applications across various industries. In pharmaceuticals, for example, quantum computers could simulate molecular interactions with unprecedented accuracy, leading to the rapid discovery of new drugs. In finance, they could optimize complex portfolios by evaluating countless scenarios simultaneously. Furthermore, in artificial intelligence, quantum computing could enhance machine learning algorithms, allowing for faster and more accurate data analysis.
Despite the promise that quantum computing holds, it is essential to acknowledge the challenges that lie ahead. Building a stable quantum computer requires maintaining qubits in their quantum states long enough to perform calculations, a feat complicated by environmental noise and decoherence. Researchers are exploring various approaches, including error correction techniques and different qubit technologies, to overcome these hurdles and bring practical quantum computing closer to reality.
The journey toward harnessing quantum computing is not merely a technological endeavor but a profound exploration of the nature of reality itself. Quantum mechanics challenges our intuitive understanding of the universe, revealing a world where particles can exist in multiple states and be interconnected in ways that defy classical logic. This exploration not only fuels scientific curiosity but also inspires a new generation of thinkers and innovators eager to unlock the secrets of the quantum realm.
As we stand on the brink of this new technological era, the amazing facts about quantum mechanics serve as a reminder of the infinite possibilities that lie ahead. The potential of quantum computing is not just a theoretical discussion; it is a pathway to solving some of humanity's most pressing challenges. With each breakthrough in our understanding of quantum mechanics, we move closer to realizing the extraordinary capabilities of quantum computers, ushering in a future where the impossible becomes possible.
Read More: Top 8 Iconic Figures Who Redefined Fashion